An Insight to the Various Applications of Hydroxyapatite
Quentin Ray Tjieh Lim ( Department of Materials Science and Engineering, National University of Singapore, Singapore 117575, Singapore. )
Xin Yi Cheng ( Department of Biomedical Engineering, National University of Singapore, Singapore 117583, Singapore. )
Chien Yi Wee ( Department of Mechanical Engineering, National University of Singapore, Singapore 117575, Singapore. )
https://doi.org/10.37155/2717-526X-0502-1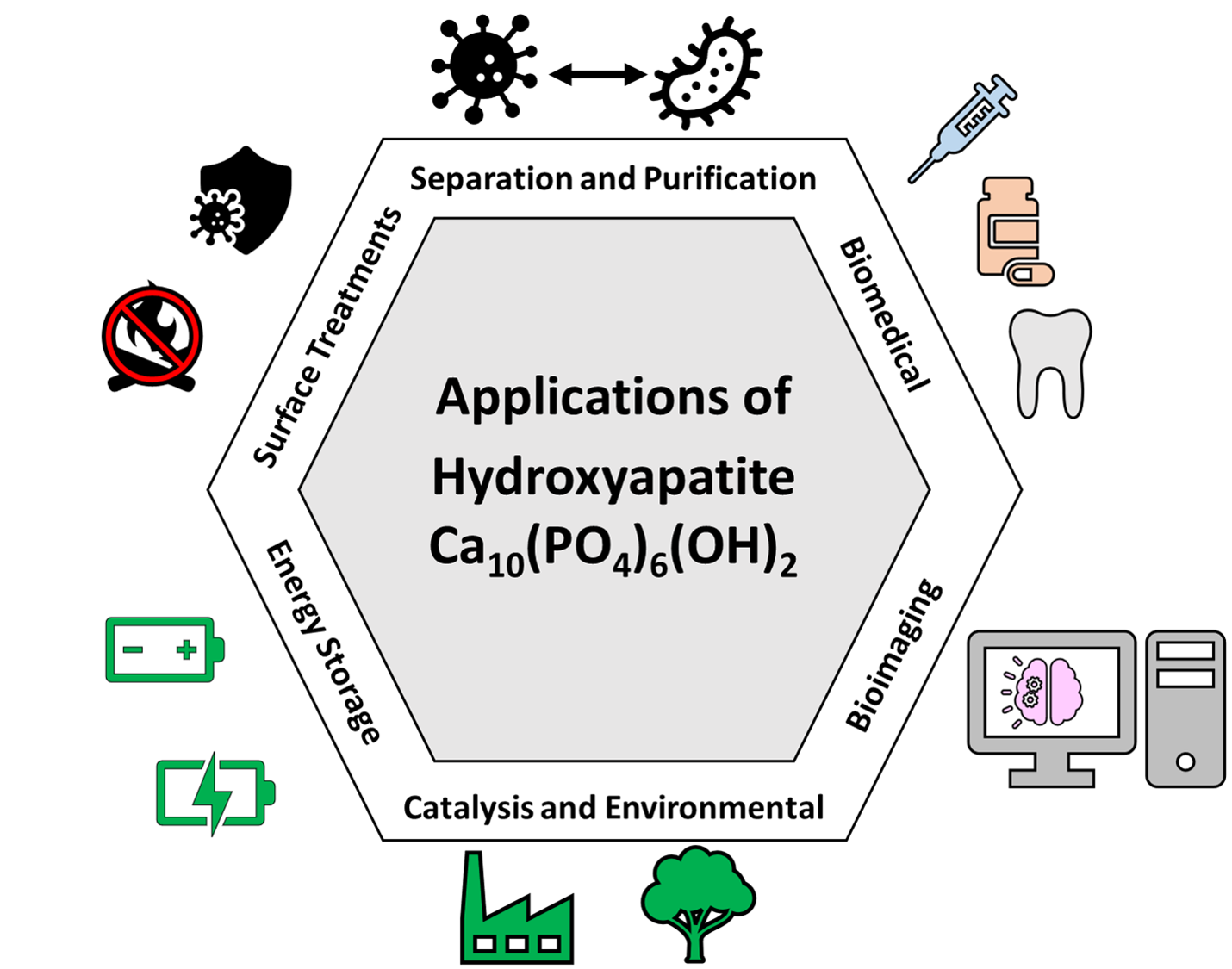
Abstract
Hydroxyapatite is a naturally occurring calcium phosphate widely used in biomedical applications because of its osteoconductivity, biocompatibility and it possesses the closest chemical similarity to natural bone. Hydroxyapatite is also a versatile material and can be used in a plethora of ways beyond biomedical applications. This review summarises the applications of hydroxyapatite in biomedical, bioimaging, separation, purification, catalysis, surface treatment and energy storage applications. Finally, this review aims to shed light on its potential for new applications of hydroxyapatite in the future.
Keywords
Hydroxyapatite; Biomedical; Bioimaging; Purification; Catalysis; Coatings; Energy storageFull Text
PDFReferences
[2] Nayak AK. Hydroxyapatite synthesis methodologies: an overview. International Journal of ChemTech Research, 2010;2(2):903-907.
[3] Wee CY, Lim QRT, Zhao Y, et al. Optimizing fabrication parameters via Taguchi method for production of high yield hydroxyapatite microsphere scaffolds using Drop‐on‐Demand inkjet method. Journal of Biomedical Materials Research Part B: Applied Biomaterials, 2023;111(11):1938-1955. https://doi.org/10.1002/jbm.b.35297
[4] Amini AR, Laurencin CT and Nukavarapu SP. Bone tissue engineering: recent advances and challenges. Critical Reviews™ in Biomedical Engineering, 2012;40(5):363-408. https://doi.org/10.1615/CritRevBiomedEng.v40.i5.10
[5] Wee CY, Yang Z and Thian ES. Past, present and future development of microspheres for bone tissue regeneration: a review. Materials Technology, 2021;36(6):364-374. https://doi.org/10.1080/10667857.2020.1759953
[6] Ribas RG, Schatkoski VM, do Amaral Montanheiro TL, et al. Current advances in bone tissue engineering concerning ceramic and bioglass scaffolds: a review. Ceramics International, 2019;45(17):21051-21061. https://doi.org/10.1016/j.ceramint.2019.07.096
[7] Jeong J, Kim JH, Shim JH, et al. Bioactive calcium phosphate materials and applications in bone regeneration. Biomaterials Research, 2019;23(1):1-11. https://doi.org/10.1186/s40824-018-0149-3
[8] Nuss KMR and von Rechenberg B. Biocompatibility issues with modern implants in bone-a review for clinical orthopedics. The Open Orthopaedics Journal, 2008;2:66-78. https://doi.org/10.2174/1874325000802010066
[9] Zhang Y, Li J, Mouser VHM, et al. Biomimetic mechanically strong one-dimensional hydroxyapatite/poly (d, l-lactide) composite inducing formation of anisotropic collagen matrix. ACS Nano, 2021;15(11):17480-17498. https://doi.org/10.1021/acsnano.1c03905
[10] Bernardo MP, da Silva BCR, Hamouda AEI, et al. PLA/Hydroxyapatite scaffolds exhibit in vitro immunological inertness and promote robust osteogenic differentiation of human mesenchymal stem cells without osteogenic stimuli. Scientific Reports, 2022;12(1):2333. https://doi.org/10.1038/s41598-022-05207-w
[11] Shuai C, Yang W, Feng P, et al. Accelerated degradation of HAP/PLLA bone scaffold by PGA blending facilitates bioactivity and osteoconductivity. Bioactive Materials, 2021;6(2):490-502. https://doi.org/10.1016/j.bioactmat.2020.09.001
[12] Alksne M, Kalvaityte M, Simoliunas E, et al. In vitro comparison of 3D printed polylactic acid/hydroxyapatite and polylactic acid/bioglass composite scaffolds: insights into materials for bone regeneration. Journal of the Mechanical Behavior of Biomedical Materials, 2020;104:103641. https://doi.org/10.1016/j.jmbbm.2020.103641
[13] Moghadasi K, Isa MSM, Ariffin MA, et al. A review on biomedical implant materials and the effect of friction stir based techniques on their mechanical and tribological properties. Journal of Materials Research and Technology, 2022;17:1054-1121. https://doi.org/10.1016/j.jmrt.2022.01.050
[14] Ripamonti U, Roden LC and Renton LF. Osteoinductive hydroxyapatite-coated titanium implants. Biomaterials, 2012;33(15):3813-3823. https://doi.org/10.1016/j.biomaterials.2012.01.050
[15] Balhuc S, Campian R, Labunet A, et al. Dental applications of systems based on hydroxyapatite nanoparticles-an evidence-based update. Crystals, 2021;11(6):674. https://doi.org/10.3390/cryst11060674
[16] O’Hagan-Wong K, Enax J, Meyer F, et al. The use of hydroxyapatite toothpaste to prevent dental caries. Odontology, 2022;110(2):223-230. https://doi.org/10.1007/s10266-021-00675-4
[17] Akhtar K, Pervez C, Zubair N, et al. Calcium hydroxyapatite nanoparticles as a reinforcement filler in dental resin nanocomposite. Journal of Materials Science: Materials in Medicine, 2021;32:1-17. https://doi.org/10.1007/s10856-021-06599-3
[18] Nasar A. Hydroxyapatite and its coatings in dental implants. Applications of Nanocomposite Materials in Dentistry. Amsterdam: Elsevier; 2019. p. 145-160.
[19] Ansari Z, Kalantar M, Kharaziha M, et al. Polycaprolactone/fluoride substituted-hydroxyapatite (PCL/FHA) nanocomposite coatings prepared by in-situ sol-gel process for dental implant applications. Progress in Organic Coatings, 2020;147:105873. https://doi.org/10.1016/j.porgcoat.2020.105873
[20] Zhao R, Yang R, Cooper PR, et al. Bone grafts and substitutes in dentistry: a review of current trends and developments. Molecules, 2021;26(10):3007. https://doi.org/10.3390/molecules26103007
[21] Meyer F, Enax J, Amaechi BT, et al. Hydroxyapatite as remineralization agent for children's dental care. Frontiers in Dental Medicine, 2022;3:24. https://doi.org/10.3389/fdmed.2022.859560
[22] Low SB, Allen EP and Kontogiorgos ED. Reduction in dental hypersensitivity with nano-hydroxyapatite, potassium nitrate, sodium monoflurophosphate and antioxidants. The Open Dentistry Journal, 2015;9:92-97. https://doi.org/10.2174/1874364101509010092
[23] Anil A, Ibraheem WI, Meshni AA, et al. Nano-hydroxyapatite (nHAp) in the remineralization of early dental caries: a scoping review. International Journal of Environmental Research and Public Health, 2022;19(9):5629. https://doi.org/10.3390/ijerph19095629
[24] Zafar MS, Khurshid Z, Najeeb S, et al. Therapeutic applications of nanotechnology in dentistry. In: Andronescu E, Grumezescu AM (editors). Nanostructures for oral medicine. Amsterdam: Elsevier; 2017. p. 833-862.
[25] Lara-Ochoa S, Ortega-Lara W and Guerrero-Beltrán CE. Hydroxyapatite nanoparticles in drug delivery: physicochemistry and applications. Pharmaceutics, 2021;13(10):1642. https://doi.org/10.3390/pharmaceutics13101642
[26] De Lama-Odría MC, Del Valle LJ and Puiggalí J. Hydroxyapatite biobased materials for treatment and diagnosis of cancer. International Journal of Molecular Sciences, 2022;23(19):11352. https://doi.org/10.3390/ijms231911352
[27] Xiong H, Du S, Ni J, et al. Mitochondria and nuclei dual-targeted heterogeneous hydroxyapatite nanoparticles for enhancing therapeutic efficacy of doxorubicin. Biomaterials, 2016;94:70-83. https://doi.org/10.1016/j.biomaterials.2016.04.004
[28] Verma R, Mishra SR, Gadore V, et al. Hydroxyapatite-based composites: excellent materials for environmental remediation and biomedical applications. Advances in Colloid and Interface Science, 2023;315:102890. https://doi.org/10.1016/j.cis.2023.102890
[29] Kong L, Mu Z, Yu Y, et al. Polyethyleneimine-stabilized hydroxyapatite nanoparticles modified with hyaluronic acid for targeted drug delivery. RSC Advances, 2016;6(104):101790-101799. https://doi.org/10.1039/C6RA19351J
[30] Chindamo G, Sapino S, Peira E, et al. Bone diseases: current approach and future perspectives in drug delivery systems for bone targeted therapeutics. Nanomaterials, 2020;10(5):875. https://doi.org/10.3390/nano10050875
[31] Maia ALC, Cavalcante CH, de Souza MGF, et al. Hydroxyapatite nanoparticles: preparation, characterization, and evaluation of their potential use in bone targeting: an animal study. Nuclear Medicine Communications, 2016;37(7):775-782. https://doi.org/10.1097/MNM.0000000000000510
[32] Mo X, Zhang D, Liu K, et al. Nano-hydroxyapatite composite scaffolds loaded with bioactive factors and drugs for bone tissue engineering. International Journal of Molecular Sciences, 2023;24(2):1291. https://doi.org/10.3390/ijms24021291
[33] Oliveira ÉR, Nie L, Podstawczyk D, et al. Advances in growth factor delivery for bone tissue engineering. International Journal of Molecular Sciences, 2021;22(2):903. https://doi.org/10.3390/ijms22020903
[34] De Witte TM, Fratila-Apachitei LE, Zadpoor AA, et al. Bone tissue engineering via growth factor delivery: from scaffolds to complex matrices. Regenerative Biomaterials, 2018;5(4):197-211. https://doi.org/10.1093/rb/rby013
[35] Tian J, Zhou H, Jiang R, et al. Preparation and biological imaging of fluorescent hydroxyapatite nanoparticles with poly (2-ethyl-2-oxazoline) through surface-initiated cationic ring-opening polymerization. Materials Science and Engineering: C, 2020;108:110424. https://doi.org/10.1016/j.msec.2019.110424
[36] Bunkoed O, Donkhampa P and Nurerk P. A nanocomposite optosensor of hydroxyapatite and graphene quantum dots embedded within highly specific polymer for norfloxacin detection. Microchemical Journal, 2020;158:105127. https://doi.org/10.1016/j.microc.2020.105127
[37] Doan VHM, Vu DD, Mondal S, et al. Yb-Gd codoped hydroxyapatite as a potential contrast agent for tumor-targeted biomedical applications. ACS Biomaterials Science & Engineering, 2023;9(8):4607-4618. https://doi.org/10.1021/acsbiomaterials.3c00383
[38] Machado TR, Leite IS, Inada NM, et al. Designing biocompatible and multicolor fluorescent hydroxyapatite nanoparticles for cell-imaging applications. Materials Today Chemistry, 2019;14:100211. https://doi.org/10.1016/j.mtchem.2019.100211
[39] Neacsu IA, Stoica AE, Vasile BS, et al. Luminescent hydroxyapatite doped with rare earth elements for biomedical applications. Nanomaterials, 2019;9(2):239. https://doi.org/10.3390/nano9020239
[40] Wan W, Li Z, Wang X, et al. Surface-fabrication of fluorescent hydroxyapatite for cancer cell imaging and bio-printing applications. Biosensors, 2022;12(6):419. https://doi.org/10.3390/bios12060419
[41] Zhang N, Lu C, Chen M, et al. Recent advances in near-infrared II imaging technology for biological detection. Journal of Nanobiotechnology, 2021;19(1):132. https://doi.org/10.1186/s12951-021-00870-z
[42] Tang W, Xu W, Zhong M, et al. Slightly doped hydroxyapatite pigments of subtractive color with high near-infrared reflectance. Journal of Solid State Chemistry, 2023;322:123947. https://doi.org/10.1016/j.jssc.2023.123947
[43] Ashokan A, Menon D, Nair S, et al. A molecular receptor targeted, hydroxyapatite nanocrystal based multi-modal contrast agent. Biomaterials, 2010;31(9):2606-2616. https://doi.org/10.1016/j.biomaterials.2009.11.113
[44] Zhang Q, Xiao L and Xiao Y. Porous nanomaterials targeting autophagy in bone regeneration. Pharmaceutics, 2021;13(10):1572. https://doi.org/10.3390/pharmaceutics13101572
[45] Chen F, Huang P, Zhu YJ, et al. The photoluminescence, drug delivery and imaging properties of multifunctional Eu3+/Gd3+ dual-doped hydroxyapatite nanorods. Biomaterials, 2011;32(34):9031-9039. https://doi.org/10.1016/j.biomaterials.2011.08.032
[46] Bai RG, Muthoosamy K, Manickam S. Nanomedicine in theranostics. In: Thomas S, Grohens Y, Ninan N (editors). Nanotechnology applications for tissue engineering. Oxford: William Andrew Publishing; 2015. p. 195-213.
[47] Lambers FM, Kuhn G and Müller R. Advances in multimodality molecular imaging of bone structure and function. BoneKEy reports, 2012;1:37. https://doi.org/10.1038/bonekey.2012.28
[48] Kargozar S, Mollazadeh S, Kermani F, et al. Hydroxyapatite nanoparticles for improved cancer theranostics. Journal of Functional Biomaterials, 2022;13(3):100. https://doi.org/10.3390/jfb13030100
[49] Villela Zumaya AL, Mincheva R, Raquez JM, et al. Nanocluster-based drug delivery and theranostic systems: towards cancer therapy. Polymers, 2022;14(6):1188. https://doi.org/10.3390/polym14061188
[50] Jo G, Park Y, Park MH, et al. Near-infrared fluorescent hydroxyapatite nanoparticles for targeted photothermal cancer therapy. Pharmaceutics, 2023;15(5):1374. https://doi.org/10.3390/pharmaceutics15051374
[51] Kharbikar BN, Zhong JX, Cuylear DL, et al. Theranostic biomaterials for tissue engineering. Current Opinion in Biomedical Engineering, 2021;19:100299. https://doi.org/10.1016/j.cobme.2021.100299
[52] Kawasaki T. Hydroxyapatite as a liquid chromatographic packing. Journal of Chromatography A, 1991;544:147-184. https://doi.org/10.1016/S0021-9673(01)83984-4
[53] Ashokan A, Rajendran V, Kumar TSS, et al. Eggshell derived hydroxyapatite microspheres for chromatographic applications by a novel dissolution-precipitation method. Ceramics International, 2021;47(13):18575-18583. https://doi.org/10.1016/j.ceramint.2021.03.183
[54] Chen G, Zhitomirsky I and Ghosh R. Fast, low-pressure chromatographic separation of proteins using hydroxyapatite nanoparticles. Talanta, 2019;199:472-477. https://doi.org/10.1016/j.talanta.2019.02.090
[55] Jing Y, Ding D, Tian G, et al. Semisynthesis of site-specifically succinylated histone reveals that succinylation regulates nucleosome unwrapping rate and DNA accessibility. Nucleic Acids Research, 2020;48(17):9538-9549. https://doi.org/10.1093/nar/gkaa663
[56] Bahri M, Hasannia S, Dabirmanesh B, et al. A multifunctional fusion peptide for tethering to hydroxyapatite and selective capture of bone morphogenetic protein from extracellular milieu. Journal of Biomedical Materials Research Part A, 2020;108(7):1459-1466. https://doi.org/10.1002/jbm.a.36915
[57] Gao S, Zheng M, Lin Y, et al. Surface‐enhanced Raman scattering analysis of serum albumin via adsorption‐exfoliation on hydroxyapatite nanoparticles for noninvasive cancers screening. Journal of Biophotonics, 2020;13(8):e202000087. https://doi.org/10.1002/jbio.202000087
[58] Lin Y, Lin J, Zheng M, et al. Quantitative and direct serum albumin detection by label-free SERS using tunable hydroxyapatite nanostructure for prostate cancer detection. Analytica Chimica Acta, 2022;1221:340101. https://doi.org/10.1016/j.aca.2022.340101
[59] Wang Y and Carta G. Competitive binding of monoclonal antibody monomer-dimer mixtures on ceramic hydroxyapatite. Journal of Chromatography A, 2019;1587:136-145. https://doi.org/10.1016/j.chroma.2018.12.023
[60] Wang Y and Carta G. Separation of monoclonal antibody monomer-dimer mixtures by gradient elution with ceramic hydroxyapatite. Journal of Chromatography A, 2020;1629:461465. https://doi.org/10.1016/j.chroma.2020.461465
[61] Alias M, Hamzah S, Saidin J, et al. Integration of hydroxyapatite from fish scales and polyethersulfone membrane for protease separation from Bacillus subtilis. Separation Science and Technology, 2022;57(6):910-928. https://doi.org/10.1080/01496395.2021.1948866
[62] Fenner F, Bachmann PA, Gibbs EPJ, et al. Structure and composition of viruses. Veterinary virology. Cambridge: Academic Press; 1987. pp. 3-19.
[63] Tleukulova Z, Stamgaliyeva Z, Dildabek A, et al. Purification of Tomato bushy stunt virus particles by one-step hydroxyapatite column chromatography. Eurasian Chemico-Technological Journal, 2021;23(4):277-282. https://doi.org/10.18321/ectj1131
[64] Kurosawa Y, Sato S, Okuyama T, et al. Sequential two-step chromatographic purification of infectious poliovirus using ceramic fluoroapatite and ceramic hydroxyapatite columns. PloS One, 2019;14(9):e0222199. https://doi.org/10.1371/journal.pone.0222199
[65] Lin MH, Li D, Tang B, et al. Defective interfering particles with broad-acting antiviral activity for Dengue, Zika, Yellow Fever, Respiratory Syncytial and SARS-CoV-2 Virus infection. Microbiology Spectrum, 2022;10(6):e03949-22. https://doi.org/10.1128/spectrum.03949-22
[66] Bharath G, Rambabu K, Hai A, et al. Synthesis of one-dimensional magnetite hydroxyapatite nanorods on reduced graphene oxide sheets for selective separation and controlled delivery of hemoglobin. Applied Surface Science, 2020;501:144215. https://doi.org/10.1016/j.apsusc.2019.144215
[67] Almulaiky YQ, Khalil NM, El-Shishtawy RM, et al. Hydroxyapatite-decorated ZrO2 for α-amylase immobilization: Toward the enhancement of enzyme stability and reusability. International Journal of Biological Macromolecules, 2021;167:299-308. https://doi.org/10.1016/j.ijbiomac.2020.11.150
[68] Coutinho TC, Tardioli PW and Farinas CS. Hydroxyapatite nanoparticles modified with metal ions for xylanase immobilization. International Journal of Biological Macromolecules, 2020;150:344-353. https://doi.org/10.1016/j.ijbiomac.2020.02.058
[69] Coutinho TC, Malafatti JOD, Paris EC, et al. Hydroxyapatite-CoFe2O4 magnetic nanoparticle composites for industrial enzyme immobilization, use, and recovery. ACS Applied Nano Materials, 2020;3(12):12334-12345. https://doi.org/10.1021/acsanm.0c02811
[70] Yamasawa R, Saito H, Yashima Y, et al. Identification, characterization, and application of a d-cysteine desulfhydrase from rice seed (Oryza sativa L.). Protein Expression and Purification, 2023;211:106341. https://doi.org/10.1016/j.pep.2023.106341
[71] Holková I, Rauová D, Mergová M, et al. Purification and product characterization of lipoxygenase from opium poppy cultures (Papaver somniferum L.). Molecules, 2019;24(23):4268. https://doi.org/10.3390/molecules24234268
[72] Rohman MM, Alam SS, Akhi AH, et al. Response of catalase to drought in barley (Hordeum vulgare L.) seedlings and its purification. African Journal of Biotechnology, 2020;19(7):478-486. https://doi.org/10.5897/AJB2020.17169
[73] Prosen H and Zupančič-Kralj L. Solid-phase microextraction. TrAC Trends in Analytical Chemistry, 1999;18(4):272-282. https://doi.org/10.1016/S0165-9936(98)00109-5
[74] Thurman EM, Mills MS. Solid-phase extraction: principles and practice. New York: Wiley; 1998.
[75] Wang J, Ni B, Li W, et al. Hydroxyapatite surface-functionalized monolithic column for selective in-tube solid phase microextraction of zoleronic acid and risedronic acid. Journal of Chromatography A, 2021;1653:462438. https://doi.org/10.1016/j.chroma.2021.462438
[76] Wang J, Li W, Xiao J, et al. Hydroxyapatite-embedded monolithic column for selective on-line solid-phase extraction of adenosine triphosphate and its phosphorylated metabolites. Journal of Chromatography B, 2019;1128:121769. https://doi.org/10.1016/j.jchromb.2019.121769
[77] Rahmani F, Hosseini MRM, Es-Haghi A, et al. A 96-monolithic inorganic hollow fiber array as a new geometry for high throughput solid-phase microextraction of doxorubicin in water and human urine samples coupled with liquid chromatography-tandem mass spectrometry. Journal of Chromatography A, 2020;1627:461413. https://doi.org/10.1016/j.chroma.2020.461413
[78] Rahimpoor R, Langari AAA, Alizadeh S, et al. Application of hydroxyapatite adsorbent packed in needle trap device for sensitive determination of trace levels of phenolic compounds in the air. Chinese Journal of Analytical Chemistry, 2021;49(12):27-35. https://doi.org/10.1016/j.cjac.2021.09.008
[79] Bayatloo MR and Nojavan S. Rapid and simple magnetic solid-phase extraction of bisphenol A from bottled water, baby bottle, and urine samples using green magnetic hydroxyapatite/β-cyclodextrin polymer nanocomposite. Microchemical Journal, 2022;175:107180. https://doi.org/10.1016/j.microc.2022.107180
[80] Lusvardi G, Malavasi G, Menabue L, et al. Removal of cadmium ion by means of synthetic hydroxyapatite. Waste Management, 2002;22(8):853-857. https://doi.org/10.1016/S0956-053X(02)00078-8
[81] Hussin MSF, Abdullah HZ, Idris MI, et al. Extraction of natural hydroxyapatite for biomedical applications-a review. Heliyon, 2022;8:e10356. https://doi.org/10.1016/j.heliyon.2022.e10356
[82] Wen T, Wu X, Liu M, et al. Efficient capture of strontium from aqueous solutions using graphene oxide-hydroxyapatite nanocomposites. Dalton Transactions, 2014;43(20):7464-7472. https://doi.org/10.1039/C3DT53591F
[83] Xiong ZC, Zhu YJ, Wang ZY, et al. Tree‐inspired ultralong hydroxyapatite nanowires‐based multifunctional aerogel with vertically aligned channels for continuous flow catalysis, water disinfection, and solar energy‐driven water purification. Advanced Functional Materials, 2022;32(9):2106978. https://doi.org/10.1002/adfm.202106978
[84] Zhang QQ, Zhu YJ, Wu J, et al. Ultralong hydroxyapatite nanowire-based filter paper for high-performance water purification. ACS Applied Materials & Interfaces, 2019;11(4):4288-4301. https://doi.org/10.1021/acsami.8b20703
[85] Koliyabandara PA, Hettithanthri O, Rathnayake A, et al. Hydroxyapatite for environmental remediation of water/wastewater. In: Kumar V, Kumar M (editors). Integrated environmental technologies for wastewater treatment and sustainable development. Amsterdam: Elsevier; 2022. p. 195-213. https://doi.org/10.1016/B978-0-323-91180-1.00004-1
[86] Jung KW, Lee SY, Choi JW, et al. A facile one-pot hydrothermal synthesis of hydroxyapatite/biochar nanocomposites: adsorption behavior and mechanisms for the removal of copper (II) from aqueous media. Chemical Engineering Journal, 2019;369:529-541. https://doi.org/10.1016/j.cej.2019.03.102
[87] El-Maghrabi HH, Younes AA, Salem AR, et al. Magnetically modified hydroxyapatite nanoparticles for the removal of uranium (VI): preparation, characterization and adsorption optimization. Journal of Hazardous Materials, 2019;378:120703. https://doi.org/10.1016/j.jhazmat.2019.05.096
[88] Wang H, Xing H, Yan K, et al. Oyster shell derived hydroxyapatite microspheres as an effective adsorbent for remediation of Coomassie brilliant blue. Advanced Powder Technology, 2022;33(2):103425. https://doi.org/10.1016/j.apt.2022.103425
[89] Azeez L, Adebisi SA, Adejumo AL, et al. Adsorptive properties of rod-shaped silver nanoparticles-functionalized biogenic hydroxyapatite for remediating methylene blue and congo red. Inorganic Chemistry Communications, 2022;142:109655. https://doi.org/10.1016/j.inoche.2022.109655
[90] Chang H, Park N, Jang Y, et al. Application of the hydroxyapatite crystallization-filtration process to recover phosphorus from wastewater effluents. Water Science and Technology, 2020;81(11):2300-2310. https://doi.org/10.2166/wst.2020.292
[91] Sun S, Gao M, Wang Y, et al. Phosphate removal via biological process coupling with hydroxyapatite crystallization in alternating anaerobic/aerobic biofilter reactor. Bioresource Technology, 2021;326:124728. https://doi.org/10.1016/j.biortech.2021.124728
[92] Bao T, Damtie MM, Yu ZM, et al. Green synthesis of Fe3O4@carbon filter media for simultaneous phosphate recovery and nitrogen removal from domestic wastewater in biological aerated filters. ACS Sustainable Chemistry & Engineering, 2019;7(19):16698-16709. https://doi.org/10.1021/acssuschemeng.9b04119
[93] Moreno EC, Gregory TM and Brown WE. Preparation and solubility of hydroxyapatite. Journal of Research of the National Bureau of Standards. Section A, Physics and Chemistry, 1968;72A(6):773-782. https://doi.org/10.6028/jres.072A.052
[94] Tounsi H, Djemal S, Petitto C, et al. Copper loaded hydroxyapatite catalyst for selective catalytic reduction of nitric oxide with ammonia. Applied Catalysis B: Environmental, 2011;107(1-2):158-163. https://doi.org/10.1016/j.apcatb.2011.07.009
[95] Kumar PA, Reddy MP, Ju LK, et al. Novel silver loaded hydroxyapatite catalyst for the selective catalytic reduction of NOx by propene. Catalysis Letters, 2008;126:78-83. https://doi.org/10.1007/s10562-008-9561-y
[96] Wei X, Wang Y, Li X, et al. Co3O4 supported on bone-derived hydroxyapatite as potential catalysts for N2O catalytic decomposition. Molecular Catalysis, 2020;491:111005. https://doi.org/10.1016/j.mcat.2020.111005
[97] Shokouhimehr M, Yek SMG, Nasrollahzadeh M, et al. Palladium nanocatalysts on hydroxyapatite: green oxidation of alcohols and reduction of nitroarenes in water. Applied Sciences, 2019;9(19):4183. https://doi.org/10.3390/app9194183
[98] Bahadorikhalili S, Arshadi H, Afrouzandeh Z, et al. Ultrasonic promoted synthesis of Ag nanoparticle decorated thiourea-functionalized magnetic hydroxyapatite: a robust inorganic-organic hybrid nanocatalyst for oxidation and reduction reactions. New Journal of Chemistry, 2020;44(21):8840-8848. https://doi.org/10.1039/D0NJ00829J
[99] Layek K. Hydroxyapatite supported gold nanoparticles catalyzed efficient reduction of nitroarenes and degradation of azo dyes. Catalysis Surveys from Asia, 2023:1-14. https://doi.org/10.1007/s10563-023-09401-2
[100] Iqbal S, ul Hassan M, Ryu HJ, et al. Environmentally benign and novel management route for radioactive corrosion products by hydroxyapatite. Journal of Nuclear Materials, 2018;507:218-225. https://doi.org/10.1016/j.jnucmat.2018.05.016
[101] Venkatesan S, ul Hassan M and Ryu HJ. Adsorption and immobilization of radioactive ionic-corrosion-products using magnetic hydroxyapatite and cold-sintering for nuclear waste management applications. Journal of Nuclear Materials, 2019;514:40-49. https://doi.org/10.1016/j.jnucmat.2018.11.026
[102] Lu K, Zhao Z, Cui J, et al. The immobilization of Sr (ll) and Co (ll) via magnetic easy-separation organophosphonate-hydroxyapatite hybrid nanoparticles. Separation and Purification Technology, 2023;315:123750. https://doi.org/10.1016/j.seppur.2023.123750
[103] Le VT, Doan VD, Nguyen DD, et al. A novel cross-linked magnetic hydroxyapatite/chitosan composite: preparation, characterization, and application for Ni (II) ion removal from aqueous solution. Water, Air, & Soil Pollution, 2018;229:1-14. https://doi.org/10.1007/s11270-018-3762-9
[104] Kim J, Sambudi NS and Cho K. Removal of Sr2+ using high-surface-area hydroxyapatite synthesized by non-additive in-situ precipitation. Journal of Environmental Management, 2019;231:788-794. https://doi.org/10.1016/j.jenvman.2018.10.100
[105] Yang K, Wang Y, Shen J, et al. Cs3Bi2I9-hydroxyapatite composite waste forms for cesium and iodine immobilization. Journal of Advanced Ceramics, 2022;11(5):712-728. https://doi.org/10.1007/s40145-021-0565-z
[106] Skwarek E, Gładysz-Płaska A, Choromańska JB, et al. Adsorption of uranium ions on nano-hydroxyapatite and modified by Ca and Ag ions. Adsorption, 2019;25:639-647. https://doi.org/10.1007/s10450-019-00063-z
[107] Zhou H, Xie Y, Wang X, et al. Efficient removal of uranium in aqueous solution by Al-doped hydroxyapatite: Static/dynamic adsorption behaviors and mechanism study. Environmental Technology & Innovation, 2022;25:102103. https://doi.org/10.1016/j.eti.2021.102103
[108] Szenknect S, Mesbah A, Descostes M, et al. Uranium removal from mining water using Cu substituted hydroxyapatite. Journal of Hazardous Materials, 2020;392:122501. https://doi.org/10.1016/j.jhazmat.2020.122501
[109] Ou T, Peng H, Su M, et al. Fast and efficient removal of uranium onto a magnetic hydroxyapatite composite: mechanism and process evaluation. Processes, 2021;9(11):1927. https://doi.org/10.3390/pr9111927
[110] Xuan K, Wang J, Gong Z, et al. Hydroxyapatite modified ZIF-67 composite with abundant binding groups for the highly efficient and selective elimination of uranium (VI) from wastewater. Journal of Hazardous Materials, 2022;426:127834. https://doi.org/10.1016/j.jhazmat.2021.127834
[111] Ji D, Wang Y, Liu Y, et al. Efficient capture of uranium by a hydroxyapatite-modified polyethyleneimine@carbon nanotube composite from radioactive nuclear waste. Dalton Transactions, 2023;52(29):10136-10144. https://doi.org/10.1039/D3DT01810E
[112] Saha S, Basu H, Rout S, et al. Nano-hydroxyapatite coated activated carbon impregnated alginate: a new hybrid sorbent for uranium removal from potable water. Journal of Environmental Chemical Engineering, 2020;8(4):103999. https://doi.org/10.1016/j.jece.2020.103999
[113] Liao J, Xiong T, Ding L, et al. Design of a renewable hydroxyapatite-biocarbon composite for the removal of uranium (VI) with high-efficiency adsorption performance. Biochar, 2022;4(1):29. https://doi.org/10.1007/s42773-022-00154-1
[114] Ahmed W, Núñez-Delgado A, Mehmood S, et al. Highly efficient uranium (VI) capture from aqueous solution by means of a hydroxyapatite-biochar nanocomposite: Adsorption behavior and mechanism. Environmental Research, 2021;201:111518. https://doi.org/10.1016/j.envres.2021.111518
[115] Su M, Tsang DCW, Ren X, et al. Removal of U (VI) from nuclear mining effluent by porous hydroxyapatite: evaluation on characteristics, mechanisms and performance. Environmental Pollution, 2019;254:112891. https://doi.org/10.1016/j.envpol.2019.07.059
[116] Wu Y, Chen D, Kong L, et al. Rapid and effective removal of uranium (VI) from aqueous solution by facile synthesized hierarchical hollow hydroxyapatite microspheres. Journal of Hazardous Materials, 2019;371:397-405. https://doi.org/10.1016/j.jhazmat.2019.02.110
[117] Ma C, Su M, Song G, et al. Fabrication of highly efficient hydroxyapatite microtubes for uranium sequestration and immobilization. Journal of Environmental Management, 2023;344:118417. https://doi.org/10.1016/j.jenvman.2023.118417
[118] Xiong T, Li Q, Liao J, et al. Highly enhanced adsorption performance to uranium (VI) by facile synthesized hydroxyapatite aerogel. Journal of Hazardous Materials, 2022;423:127184. https://doi.org/10.1016/j.jhazmat.2021.127184
[119] Huang S, Chen C, Zhao Z, et al. Highly efficient separation of uranium from wastewater by in situ synthesized hydroxyapatite modified coal fly ash composite aerogel. Journal of Industrial and Engineering Chemistry, 2023;118:418-431. https://doi.org/10.1016/j.jiec.2022.11.026
[120] Xiong T, Li Q, Liao J, et al. Design of hydroxyapatite aerogel with excellent adsorption performance to uranium. Journal of Environmental Chemical Engineering, 2021;9(6):106364. https://doi.org/10.1016/j.jece.2021.106364
[121] Wang Y, Chen B, Xiong T, et al. Highly efficient uranium capture from wastewater by hydroxyapatite aerogels prepared with konjac gum as template. Journal of Water Process Engineering, 2022;48:102919. https://doi.org/10.1016/j.jwpe.2022.102919
[122] Xiong T, Jia L, Li Q, et al. Efficient removal of uranium by hydroxyapatite modified kaolin aerogel. Separation and Purification Technology, 2022;299:121776. https://doi.org/10.1016/j.seppur.2022.121776
[123] Park JH, Lee GD, Nishikata A, et al. Anticorrosive behavior of hydroxyapatite as an environmentally friendly pigment. Corrosion Science, 2002;44(5):1087-1095. https://doi.org/10.1016/S0010-938X(01)00118-4
[124] Guo XJ, Yuan XY, Zhao SR, et al. Improving anticorrosion performance of hydroxyapatite via controlling exposed crystal surface and applications. Journal of Alloys and Compounds, 2020;845:156290. https://doi.org/10.1016/j.jallcom.2020.156290
[125] Cheng L, Wu H, Li J, et al. Polydopamine modified ultrathin hydroxyapatite nanosheets for anti-corrosion reinforcement in polymeric coatings. Corrosion Science, 2021;178:109064. https://doi.org/10.1016/j.corsci.2020.109064
[126] Zhou Z, Zheng B, Gu Y, et al. New approach for improving anticorrosion and biocompatibility of magnesium alloys via polydopamine intermediate layer-induced hydroxyapatite coating. Surfaces and Interfaces, 2020;19:100501. https://doi.org/10.1016/j.surfin.2020.100501
[127] Guo Y, Yu Z, Chen L, et al. Preparation and performance of a composite epoxy coating based on modified hydroxyapatite. Surface and Coatings Technology, 2022;443:128614. https://doi.org/10.1016/j.surfcoat.2022.128614
[128] Xu X, Wang H, Wu J, et al. Hydrothermal in-situ synthesis and anti-corrosion performance of zinc oxide hydroxyapatite nanocomposite anti-corrosive pigment. Coatings, 2022;12(4):420. https://doi.org/10.3390/coatings12040420
[129] Xue XZ, Zhang JY, Zhou D, et al. In-situ bonding technology and excellent anticorrosion activity of graphene oxide/hydroxyapatite nanocomposite pigment. Dyes and Pigments, 2019;160:109-118. https://doi.org/10.1016/j.dyepig.2018.07.057
[130] Predoi D, Popa CL, Chapon P, et al. Evaluation of the antimicrobial activity of different antibiotics enhanced with silver-doped hydroxyapatite thin films. Materials, 2016;9(9):778. https://doi.org/10.3390/ma9090778
[131] Trentin DS, Silva DB, Frasson AP, et al. Natural green coating inhibits adhesion of clinically important bacteria. Scientific Reports, 2015;5(1):8287. https://doi.org/10.1038/srep08287
[132] Sinulingga K, Sirait M, Siregar N, et al. Investigation of antibacterial activity and cell viability of Ag/Mg and Ag/Zn co-doped hydroxyapatite derived from natural limestone. ACS Omega, 2021;6(49):34185-34191. https://doi.org/10.1021/acsomega.1c05921
[133] Esmaeili Y, Zamindar N and Mohammadi R. The effect of polypropylene film containing nano-hydroxyapatite on Physicochemical and microbiological properties of button mushrooms (Agaricus bisporus) under Modified atmosphere packaging. Journal of Food Measurement and Characterization, 2023;17(1):773-786. https://doi.org/10.1007/s11694-022-01613-w
[134] Yin IX, Zhang J, Zhao IS, et al. The antibacterial mechanism of silver nanoparticles and its application in dentistry. International Journal of Nanomedicine, 2020;15:2555-2562. https://doi.org/10.2147/IJN.S246764
[135] Feng QL, Wu J, Chen GQ, et al. A mechanistic study of the antibacterial effect of silver ions on Escherichia coli and Staphylococcus aureus. Journal of Biomedical Materials Research, 2000;52(4):662-668.
[136] Gökmen FÖ. Hydroxyapatite-doped polyhydroxyethylmethacrylate hydrogels as smart porous packaging materials. Food and Bioprocess Technology, 2023;16:2692-2704. https://doi.org/10.1007/s11947-023-03097-y
[137] Malvano F, Montone AMI, Capparelli R, et al. Development of a novel active edible coating containing hydroxyapatite for food shelf-life extension. Chemical Engineering Transactions, 2021;87:25-30. https://doi.org/10.3303/CET2187005
[138] Malvano F, Montone AMI, Capuano F, et al. Effects of active alginate edible coating enriched with hydroxyapatite-quercetin complexes during the cold storage of fresh chicken fillets. Food Packaging and Shelf Life, 2022;32:100847. https://doi.org/10.1016/j.fpsl.2022.100847
[139] Wang Y, Cheng M, Yan X, et al. Preparation and characterization of an active packaging film loaded with tea tree oil-hydroxyapatite porous microspheres. Industrial Crops and Products, 2023;199:116783. https://doi.org/10.1016/j.indcrop.2023.116783
[140] Akaki T, Maehara H and Tooyama M. Development of wood and wood ash-based hydroxyapatite composites and their fire-retarding properties. Journal of Wood Science, 2012;58(6):532-537. https://doi.org/10.1007/s10086-012-1276-4
[141] Tonsuaadu K, Gross KA, Plūduma L, et al. A review on the thermal stability of calcium apatites. Journal of Thermal Analysis and Calorimetry, 2012;110(2):647-659. https://doi.org/10.1007/s10973-011-1877-y
[142] Zhu YJ. Multifunctional fire-resistant paper based on ultralong hydroxyapatite nanowires. Chinese Journal of Chemistry, 2021;39(8):2296-2314. https://doi.org/10.1002/cjoc.202100170
[143] Chen FF, Zhu YJ, Chen F, et al. Fire alarm wallpaper based on fire-resistant hydroxyapatite nanowire inorganic paper and graphene oxide thermosensitive sensor. ACS Nano, 2018;12(4):3159-3171. https://doi.org/10.1021/acsnano.8b00047
[144] Vahabi H, Gholami F, Karaseva V, et al. Novel nanocomposites based on poly (ethylene-co-vinyl acetate) for coating applications: the complementary actions of hydroxyapatite, MWCNTs and ammonium polyphosphate on flame retardancy. Progress in Organic Coatings, 2017;113:207-217. https://doi.org/10.1016/j.porgcoat.2017.08.009
[145] Ai J, Guo Z and Liu W. Superamphiphobic coatings with antifouling and nonflammable properties using functionalized hydroxyapatite. New Journal of Chemistry, 2021;45(14):6238-6246. https://doi.org/10.1039/D1NJ00277E
[146] Członka S, Kairytė A, Miedzińska K, et al. Polyurethane hybrid composites reinforced with lavender residue functionalized with kaolinite and hydroxyapatite. Materials, 2021;14(2):415. https://doi.org/10.3390/ma14020415
[147] Nihmath A and Ramesan MT. Studies on the role of hydroxyapatite nanoparticles in imparting unique thermal, dielectric, flame retardancy and petroleum fuel resistance to novel chlorinated EPDM/chlorinated NBR blend. Research on Chemical Intermediates, 2020;46(11):5049-5068. https://doi.org/10.1007/s11164-020-04239-z
[148] Zhou Y, Qiu S, Ding L, et al. Innovative design of environmentally friendly silicon-based polyphosphazene-functionalized hydroxyapatite nanowires: an efficient enhancement strategy for the fire safety and mechanical properties of unsaturated polyester. Chemical Engineering Journal, 2022;437:135489. https://doi.org/10.1016/j.cej.2022.135489
[149] Huang Q, Xue H, Dong R, et al. Simple and green synthesis of calcium alginate/hydroxyapatite hybrid material without high temperature treatment and its flame retardancy. Cellulose, 2022;29(3):1759-1774. https://doi.org/10.1007/s10570-021-04373-w
[150] Zhu J, Li X, Li D, et al. Thermal insulation and flame retardancy of the hydroxyapatite nanorods/sodium alginate composite aerogel with a double-crosslinked structure. ACS Applied Materials & Interfaces, 2022;14(40):45822-45831. https://doi.org/10.1021/acsami.2c12254
[151] Zhu J, Xiong R, Zhao F, et al. Lightweight, high-strength, and anisotropic structure composite aerogel based on hydroxyapatite nanocrystal and chitosan with thermal insulation and flame retardant properties. ACS Sustainable Chemistry & Engineering, 2019;8(1):71-83. https://doi.org/10.1021/acssuschemeng.9b03953
[152] Nabipour H, Wang X, Song L, et al. A fully bio-based coating made from alginate, chitosan and hydroxyapatite for protecting flexible polyurethane foam from fire. Carbohydrate Polymers, 2020;246:116641. https://doi.org/10.1016/j.carbpol.2020.116641
[153] Wang ZY, Zhu YJ, Chen YQ, et al. Flexible nanocomposite paper with superior fire retardance, mechanical properties and electrical insulation by engineering ultralong hydroxyapatite nanowires and aramid nanofibers. Chemical Engineering Journal, 2022;444:136470. https://doi.org/10.1016/j.cej.2022.136470
[154] Huang J, E S, Si L, et al. Composite films of hydroxyethyl cellulose and hydroxyapatite nanowires with high mechanical strength and electrical insulation property. Journal of Wood Chemistry and Technology, 2022;42(1):15-25. https://doi.org/10.1080/02773813.2021.1998128
[155] Dong LY, Zhu YJ and Wu J. Wet end chemical properties of a new kind of fire-resistant paper pulp based on ultralong hydroxyapatite nanowires. Molecules, 2022;27(20):6808. https://doi.org/10.3390/molecules27206808
[156] Li H, Wu D, Wu J, et al. Flexible, high-wettability and fire-resistant separators based on hydroxyapatite nanowires for advanced lithium-ion batteries. Advanced Materials, 2017;29(44):1703548. https://doi.org/10.1002/adma.201703548
[157] Wen J, Zhang R, Zhao Q, et al. Hydroxyapatite nanowire-reinforced poly (ethylene oxide)-based polymer solid electrolyte for application in high-temperature lithium batteries. ACS Applied Materials & Interfaces, 2020;12(49):54637-54643. https://doi.org/10.1021/acsami.0c15692
[158] Liang Y, Liu Y, Chen D, et al. Hydroxyapatite functionalization of solid polymer electrolytes for high-conductivity solid-state lithium-ion batteries. Materials Today Energy, 2021;20:100694. https://doi.org/10.1016/j.mtener.2021.100694
[159] Xi C, Cui X, Zhang R, et al. Utilizing an oxygen-rich interface by hydroxyapatite to regulate the linear diffusion for the stable solid-state electrolytes. ACS Applied Materials & Interfaces, 2022;14(29):33392-33399. https://doi.org/10.1021/acsami.2c09207
[160] Rao Z, Yang Z, Gong W, et al. Simultaneously suppressing lithium dendrite growth and Mn dissolution by integration of a safe inorganic separator in a LiMn2O4/Li battery. Journal of Materials Chemistry A, 2020;8(7):3859-3864. https://doi.org/10.1039/C9TA12979K
[161] Li R, Sun X, Zou J, et al. Hydroxyapatite nanowires composite interlayer based on aramid fiber paper for Li-S batteries. Journal of Electroanalytical Chemistry, 2020;856:113662. https://doi.org/10.1016/j.jelechem.2019.113662
[162] Wang JY, Tong XF, Peng QF, et al. Efficient interface enabled by nano-hydroxyapatite@porous carbon for lithium-sulfur batteries. Journal of Electrochemistry, 2022;28(11):2219008.
[163] Saroja APVK, Kumar A, Moharana BC, et al. Design of porous calcium phosphate based gel polymer electrolyte for quasi-solid state sodium ion battery. Journal of Electroanalytical Chemistry, 2020;859:113864. https://doi.org/10.1016/j.jelechem.2020.113864
[164] Rao Z, Meng J, Wu J, et al. A multifunctional inorganic composite separator for stable high-safety lithium-sulfur batteries. ACS Applied Energy Materials, 2020;3(10):10139-10146. https://doi.org/10.1021/acsaem.0c01842
[165] Hou Y, Huang Z, Chen Z, et al. Bifunctional separators design for safe lithium-ion batteries: suppressed lithium dendrites and fire retardance. Nano Energy, 2022;97:107204. https://doi.org/10.1016/j.nanoen.2022.107204
[166] Liu Y, Li C, Li C, et al. Highly thermally stable, highly electrolyte-wettable hydroxyapatite/cellulose nanofiber hybrid separators for lithium-ion batteries. ACS Applied Energy Materials, 2023;6(7):3862-3871. https://doi.org/10.1021/acsaem.2c04170
[167] Chen W, Wang X, Liang J, et al. A high performance polyacrylonitrile composite separator with cellulose acetate and nano-hydroxyapatite for lithium-ion batteries. Membranes, 2022;12(2):124. https://doi.org/10.3390/membranes12020124
[168] Selvam S and Yim JH. High temperature-functioning ceramic-based ionic liquid electrolyte engraved planar HAp/PVP/MnO2@MnCO3 supercapacitors on carbon cloth. Journal of Materials Chemistry A, 2021;9(25):14319-14330. https://doi.org/10.1039/D0TA11980F
[169] Eswaran M, Swamiappan S, Chokkiah B, et al. A green and economical approach to derive nanostructured hydroxyapatite from Garra mullya fish scale waste for biocompatible energy storage applications. Materials Letters, 2021;302:130341. https://doi.org/10.1016/j.matlet.2021.130341
[170] Chu M, Zhai Y, Shang N, et al. Functions of hydroxyapatite in fabricating N-doped carbon for excellent catalysts and supercapacitors. Catalysis Science & Technology, 2019;9(18):4952-4960. https://doi.org/10.1039/C9CY00804G
[171] Chu M, Zhai Y, Shang N, et al. N-doped carbon derived from the monomer of chitin for high-performance supercapacitor. Applied Surface Science, 2020;517:146140. https://doi.org/10.1016/j.apsusc.2020.146140
[172] Lv S, Ma L, Zhou Q, et al. One-step pyrolysis toward nitrogen-doped hierarchical porous carbons for supercapacitors. Journal of Materials Science, 2020;55:12191-12202. https://doi.org/10.1007/s10853-020-04876-0
[173] Li WC, Chen RK and Wen TC. The role of nano-hydroxyapatite bearing zwitterion within carboxylated chitosan hydrogel electrolyte in improving supercapacitor performance. Electrochimica Acta, 2023;466:143064. https://doi.org/10.1016/j.electacta.2023.143064
[174] Roveri N, Falini G, Sidoti MC, et al. Biologically inspired growth of hydroxyapatite nanocrystals inside self-assembled collagen fibers. Materials Science and Engineering: C, 2003;23(3):441-446. https://doi.org/10.1016/S0928-4931(02)00318-1
[175] Sadek H, Siddique SK, Wang CW, et al. Bioinspired nanonetwork hydroxyapatite from block copolymer templated synthesis for mechanical metamaterials. ACS Nano, 2022;16(11):18298-18306. https://doi.org/10.1021/acsnano.2c06040
[176] Bai H, Walsh F, Gludovatz B, et al. Bioinspired hydroxyapatite/poly (methyl methacrylate) composite with a nacre-mimetic architecture by a bidirectional freezing method. Advanced Materials, 2016;28(1):50-56. https://doi.org/10.1002/adma.201504313
[177] Qi Y, Cheng Z, Ye Z, et al. Bioinspired mineralization with hydroxyapatite and hierarchical naturally aligned nanofibrillar cellulose. ACS Applied Materials & Interfaces, 2019;11(31):27598-27604. https://doi.org/10.1021/acsami.9b09443
[178] Huang C, Fang G, Zhao Y, et al. Bio-inspired nanocomposite by layer-by-layer coating of chitosan/hyaluronic acid multilayers on a hard nanocellulose-hydroxyapatite matrix. Carbohydrate Polymers, 2019;222:115036. https://doi.org/10.1016/j.carbpol.2019.115036
[179] Wan F, Ping H, Wang W, et al. Hydroxyapatite-reinforced alginate fibers with bioinspired dually aligned architectures. Carbohydrate Polymers, 2021;267:118167. https://doi.org/10.1016/j.carbpol.2021.118167
[180] Gomez-Vazquez OM, Correa-Piña BA, Zubieta-Otero LF, et al. Synthesis and characterization of bioinspired nano-hydroxyapatite by wet chemical precipitation. Ceramics International, 2021;47(23):32775-32785. https://doi.org/10.1016/j.ceramint.2021.08.174
[181] Fu XK, Cao HB, An YL, et al. Bioinspired hydroxyapatite coating infiltrated with a graphene oxide hybrid supramolecular hydrogel orchestrates antibacterial and self-lubricating performance. ACS Applied Materials & Interfaces, 2022;14(28):31702-31714. https://doi.org/10.1021/acsami.2c07869
[182] Mu Y, Feng H, Zhang S, et al. Development of highly permeable and antifouling ultrafiltration membranes based on the synergistic effect of carboxylated polysulfone and bio-inspired co-deposition modified hydroxyapatite nanotubes. Journal of Colloid and Interface Science, 2020;572:48-61. https://doi.org/10.1016/j.jcis.2020.03.072
[183] Shao CS, Chen LJ, Tang RM, et al. Polarized hydroxyapatite/BaTiO3 scaffolds with bio-inspired porous structure for enhanced bone penetration. Rare Metals, 2022;41(1):67-77. https://doi.org/10.1007/s12598-021-01798-x
[184] Platini M, Ropars T, Pelletier B, et al. CPU overheating prediction in HPC systems. Concurrency and Computation: Practice and Experience, 2021;33(13):e6231. https://doi.org/10.1002/cpe.6231
[185] Lee M and Kim H. COVID-19 pandemic and microplastic pollution. Nanomaterials, 2022;12(5):851. https://doi.org/10.3390/nano12050851
[186] Romagnoli L and Fanelli RM. Annual food waste per capita as influenced by geographical variations. Rivista Di Studi Sulla Sostenibilità, 2019. pp. 59-76.
Copyright © 2023 Quentin Ray Tjieh Lim, Xin Yi Cheng, Chien Yi Wee

This work is licensed under a Creative Commons Attribution 4.0 International License